
Home

Students

Research activities

Publications

Contact

Links

|
|
Research activities
Intelligent Transportation Systems
Fault Diagnosis of Complex Engineering Systems: Applications to Autonomous Vehicles
Flight formation and control of Unmanned Aerial Vehicles (UAVs), with applications to Precision agriculture
Active Magnetic Bearing Control Laws and their Applications in Flywheel Energy Storage Systems
Intelligent Transportation Systems
In this research we are developing a guidance system which will allow
autonomous car driving on highways, and traffic management. The system is
based on a distributed wireless network of sensors and controllers, which are a
part of the road infrastructure. The required modifications for standard vehicles,
so they can have the ability of autonomous driving by this developed approach,
are minimal. The driver with the modified car will have the choice, to drive his
car manually, or to be driven by the system, autonomously. This system will
allow standard unmodified vehicles (manually driven) and modified cars
(autonomously or manually driven) on the same road. It is based, as much as
possible, on the road infrastructure (with no need for onboard expensive devices
such DGPS or a LIDAR). As part of this research, we are developing a test-bed
with a miniature road and miniature electric cars (scale 1:10 compared to a real
road and cars). Using this facility, we are able to demonstrate our developed
cooperative-control approaches, such as the coordinated path-following methods
we have presented in [20], [31] and [32] (see the list of Refereed chapters in
collective volumes and Conference proceedings). In the Refereed Article in
Scientific Journal [8], we showed a new control strategy for autonomous road
tracking that is based on optimal control principles and LMI (linear matrix
inequalities) formulation. A vehicle yaw stability controller that utilizes an active
limited slip differential has been developed and presented in the Refereed Article
in Scientific Journal [15].
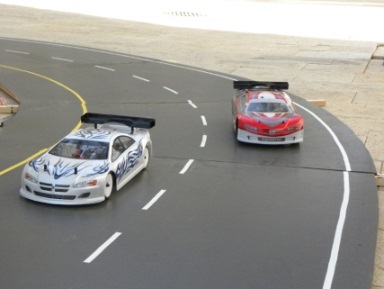
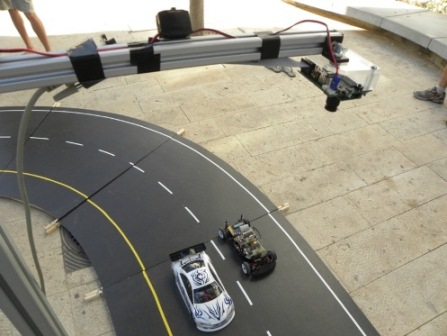
Back to top
Fault Diagnosis of Complex Engineering Systems: Applications to
Autonomous Vehicles
In this research, we are developing algorithms for fault detection, isolation
and identification, which are applicable to systems with hybrid dynamics (i.e.,
dynamics that combine continuous and discrete states). We are using the model-based
approach, where a dynamical model of the normal (healthy) system is utilized to develop
condition-indexes called residuals. These residuals measure the consistency between
the model and the real system (in real time). We have developed a new health monitoring
approach based on a special dynamical model called hybrid bond graph (HBG) (see the
Authored book [1] and the Refereed Articles in Scientific Journals [4], [5], [6], [7], [10]).
From this model, we can systematically generate global residuals (called global analytical
redundancy relations - GARRs) which represent consistency in a global sense (meaning, these
relations are relevant to all system modes). The method has been implemented for health
monitoring of the hydraulic steering system of an autonomous mobile robot (in the Refereed
Article in Scientific Journals [9]). Our recent efforts are devoted to the integration of
the HBG approach with diagnostic tools and methods from the theory of discrete event systems
(i.e., systems with discrete dynamics only), and the applications of these health monitoring
methods to autonomous vehicles. This part of the research was supported by the Israel Science
Foundation (ISF). Important results were published in two articles (Refereed Articles in Scientific Journals [11] and [14]).
The method has been implemented for health monitoring of the hydraulic
steering systems of an autonomous mobile robot (see a photo below).
Back to top
Flight formation and control of Unmanned Aerial Vehicles (UAVs), with applications to Precision agriculture
The development of multi-agent systems is of considerable interest to the control community over the last years.
Such systems integrate several, physically independent agents, that share a common goal. This interest is due to
the broad range of applications of multi-agent systems in various domains, including undersea, land, air and space.
Our goal in this research is to allow efficient coordination and control of multiple UAVs (unmanned aerial vehicles).
Current efforts are devoted to the problem of outdoor flight formation of miniature rotorcrafts, subject to wind
disturbances. The goal is to keep relative position between UAVs of less than 1m, while the complete formation is
required to follow a desired spatial trajectory. Our current results, have been presented in [18], [25] and [24]
(see the list of Refereed chapters in collective volumes and Conference proceedings), and published in the Refereed
Article in Scientific Journal [12]. We also develop new applications of UAVs in the context of precision agriculture.
Under a research funded by the ABC Robotics Initiative we study the problem of robotic tomato pollination in greenhouses
by a group of drones.
Back to top
Active Magnetic Bearing Control Laws and their Applications in Flywheel Energy Storage Systems
One of the promising eco-friendly alternatives to efficient storage of energy is based on flywheels. First flywheel
energy storage systems (FESS) have been based on ball bearings to support the rotating flywheel. Due to the large
mechanical friction of such bearings, these storage systems suffered from very low efficiency. Moreover, industrial
ball bearings require maintenance and lubrication. An active magnetic bearing (AMB) utilizes a strong magnetic field
to support a rotating shaft with zero mechanical friction. The AMB eliminates energy losses due to mechanical friction,
but requires closed loop control and consumes electrical energy. In this research, we are developing optimal magnetic
bearings, from both, mechanical design and control, point of views. Our goal is to minimize energy losses and to
increase the storage system efficiency. The AMB is a highly nonlinear and unstable system. The air gap (between
the rotor and the stator) is of the order of 200-300 micron and the targeted rotating speed is 60,000 RPM.
In addition to the electromagnetic and mechanical design, robust linear and nonlinear control algorithms
for AMBs were designed and tested. Some of our early results regarding control systems for AMB were presented
in the Refereed chapters in collective volumes and Conference proceedings [19], [22], [27], [28], [29], [30].
This research was supported by the planning and budgeting committee of the Israel council for higher education
(under the VATAT-NRCN research grant). Our current research efforts are devoted to the use of AMB technology in
applications that require (sub-micron) 6-DOF precise motion. Our approach is based on the principles of electromagnetic
levitation and adaptive control algorithms. Recent results were summarized in a Refereed Article in Scientific Journal
(see article [16]).
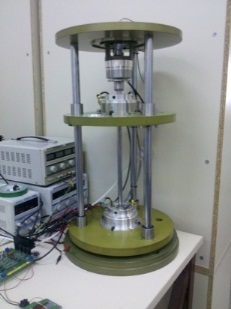
Back to top
Free website templates
|
|